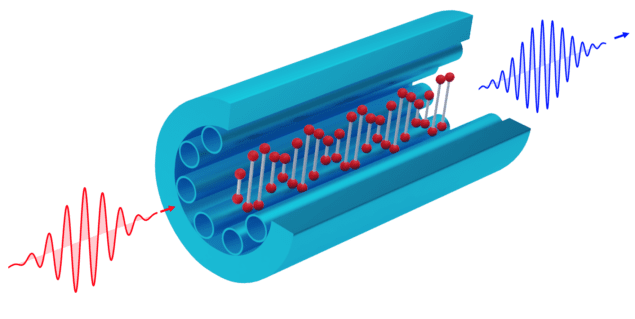
A new technique that keeps two photons entangled while greatly increasing the frequency of one of them has been demonstrated by researchers in Germany. The work could prove useful in quantum computation, imaging and quantum communications.
Two particles are considered entangled if their quantum states are correlated to such an extent that they cannot be described independently. This property is central to many areas of quantum technology, including schemes that use photons to exchange keys in quantum cryptography. Entanglement is, however, fragile, meaning that changing the frequency of one photon risks destroying its entanglement with another.
This is a problem because being able to perform such frequency shifts could be very useful. “At telecommunications wavelengths – around 1.5 microns – it’s actually quite difficult to get good quantum detectors,” explains optical physicist Philip Russell of the Max Planck Institute for the Science of Light (MPL) in Erlangen. “If you want to detect single photons, you’d like to do it in the visible where the detectors are much more sensitive and have much less noise.” Russell adds that while an ordinary telecom modulator can shift the frequency by a few gigahertz while preserving entanglement, shifting a photon from the telecom range into the visible requires a frequency shift of hundreds of terahertz (THz).
Phonon–photon effect
In the new work, MPL researchers led by David Novoa developed a technique that involves sending pulses of 1064 nm laser light through a photonic crystal fibre filled with pressurized hydrogen. Before each pulse enters the fibre, the team diverts a small fraction of it to produce highly-entangled pairs of photons – one at 1425 nm and one at 849 nm – via a well-understood non-linear optics process. The remainder of each 1064 nm light pulse then passes through the hydrogen gas, where it produces quantized excitations called phonons. While the 849 nm photon is sent straight to a detector, its entangled partner at 1425 nm instead passes through the fibre, where it exchanges energy with the phonons.
As Russell explains, this configuration produces a non-linear effect that acts like a diffraction grating in the hydrogen gas – with one important difference. “It’s a periodic modulation of the refractive index in space, and it’s travelling at a substantial fraction of the speed of light,” he says. When a single photon passes through this “diffraction grating”, therefore, it does not simply change direction, as it would if it passed through a static diffraction grating. Instead, it is either significantly red- or blue-shifted by its motion relative to the grating. The shifting process is relatively noise free, allowing the team to use it to shift the 1425 nm photons up to 894 nm before sending them to the detector.
When the researchers measured the quantum correlations between these 894 nm photons and the “untouched” 849 nm ones, they found they were still entangled despite the former undergoing an increase in frequency of 125 THz. “The key that made this possible – and this was done about 10 years ago in my group – was the realization that in these hollow-core fibres we have the unique ability to engineer the wavelength at which the pulses don’t disperse by changing the pressure of the gas,” Russell explains. “We can create an optical phonon…and then we can re-use that phonon in a completely different part of the spectrum.”
Efficiency savings
In addition to the extraordinarily large increase in frequency, the new system has several advantages over established frequency-shifting rivals such as nonlinear crystals. First, the efficiency of the process is 70–80%, which is almost unprecedented for single photons. Moreover, the researchers believe that by varying the frequency of the incoming laser beam, the pressure of the gas in the fibre or the nature of the gas itself, it should be relatively trivial to alter the phonon frequency, and thereby to alter the amount by which an incoming photon’s frequency is increased. “Everything is reconfigurable,” explains Russell’s colleague Nicolas Joly. “You don’t need to change anything on the optical table – you just change the bottle of gas underneath.”
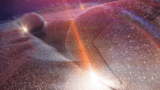
Frequency upconversion makes infrared light visible
The MPL team is part of a major German national collaboration to develop systems for quantum key distribution, and Russell says that other members of the collaboration have discussed using the team’s technology for quantum networking and quantum key distribution. “We’re doing the kind of blue skies, experimental, curiosity-driven end of this project,” Russell explains. “This result might well be of interest to them.”
Alexei Sokolov, a laser physicist at Texas A&M University in the US who was not involved in the research, calls it an important proof of principle. “Now we know that this can be done, if there is a need for quantum communication systems that use light at different wavelengths, we know how to interface them.” He also believes the work could find applications in entangled-photon spectroscopy and microscopy, which is used to create accurate images in low-intensity light: “You may want to use adjustable frequencies of your probe photons,” he suggests.
The research is published in Science.
- The paragraph beginning “As Russell explains…” has been modified since publication to reflect a clarification from Russell regarding his comments on noise in the shifting process.